Georgina A.
Rivera-Ingraham
Introduction
But... what is the intertidal and what type of organisms does it host?
The intertidal zone (also called littoral zone), is the area of the coast that is above the water line at low tide and under the water at high tide.
Intertidal animals therefore live at the margins of the marine and terrestrial realms, and must daily cope with the most extreme changes in environmental conditions.
But many of the intertidal organisms have limited or no possibility of movement, and when low tide comes, they are exposed to these extreme conditions without being able to find refuge. They can only rely on their specific adaptations to endure the hard conditions they are exposed to during emmersion.
Limpets are a good example. They mobility is limited to the rock they inhabit. During low tide they not only have to endure low oxygen conditions (hypoxia), but also high temperatures and radiation during the summer and freezing temperatures during the winter season. So how do they do it? Lets see some examples...
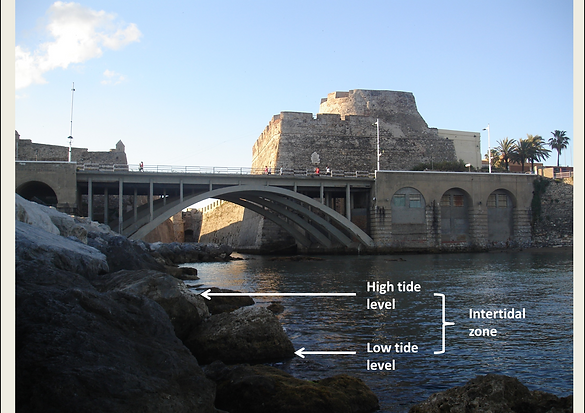

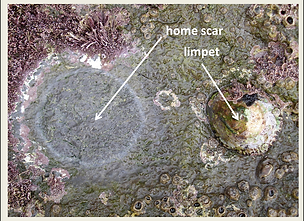
BEHAVIORAL ADAPTATIONS
The ferruginean limpet (left) has adopted a very interesting way to avoid desiccation. Animals during their life, grow their shells accordingly to the microtopography of a very specific area of the rock, which constitutes its home (or "home scar") (right). During low tide, animals are always firmely pressed on the surface of their home scar, leaving no air gaps between the shell and the rock's surface, and preventing desiccation. Only during high tide, when there is no risk for desiccation, animals leave their home scar to search for food.
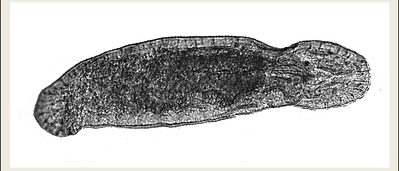
PHYSIOLOGICAL ADAPTATIONS
The intertidal platyhelminth Macrostomum lignano (left), is a 1mm long flatworm that inhabits the sands of the Mediterranean sea. It has numerous physiological mechanisms that allow them to withstand a wide variety of environmental changes that occur in the intertidal. One good example is how these animals are able to decrease their respiration rates and enter a state of metabolic depression when oxygen levels are low, allowing them to enhance their chance of survival under these hypoxic conditions.
But the examples could go on an on, and greatly vary among species. But the next question that would arise, is...
Why study these organisms and what information can they provide us?
Climate change is one of the greatest environmental, social and economic threats our planet is facing. If you want to read a simple and summarized view regarding climate change, its causes and consequences, you can go here.
The impact of climate change on coastal areas is attracting much research, since there is strong scientific consensus that suggests that coastal marine systems are highly threatened by anthropogenic global climate change. One of the most interesting sentry organisms for studying climate change are those regularly exposed to environmental changes, such as coastal, estuarine and lagoon media, and this is the main reason why my research is focused on organisms inhabiting these areas. Increased greenhouse gas concentrations will ultimately derive in significant changes in water salinity, pH and oxygenation (see figure below). The impact that climate change will have on these organisms will be a key-importance knowledge, not only to realistically evaluate how it will affect the ecosystems in terms of biodiversity, but also due to the economic importance of these habitats: it has been estimated that the intertidal zone out to the continental shelf provides over US$ 14 trillion worth of goods and services per year, which would be equivalent to 43% of the global total.
In this context, understanding the ecophysiological adaptation of organisms is essential for realistically evaluating the impact that changes in environmental conditions will have on organisms and ecosystems.
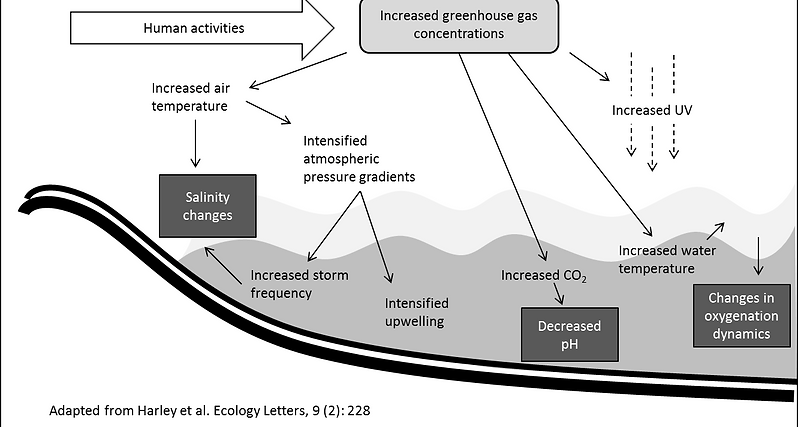
There are many ways to approach this study, but I am mainly interested in an oxidative-stress approach. ¿What does that mean?
Reactive oxygen and nitrogen species... compounds which are produced as a consequence of stress exposure and which may be dangerous to cells.
A common consequence of most abiotic and biotic stresses is that they result, at some stage of stress exposure, in an increased production of free radicals (FR), reactive-oxygen species (ROS) and reactive-nitrogen species (RNS) that occur in aerobically functioning cells as by-products of cellular respiration and of several cellular defense and detoxification mechanisms. ROS and RNS in high concentrations can constitute a threat to the viability of the cells. Generally, cells fight ROS production through the activity of several enzymes which can transform ROS into less dangereous products or though other antioxidant compounds. But the accumulation of ROS that cannot be detoxified by the cellular antioxidant system leads to “oxidative stress” (OS, induced by ROS) (Sies, 1985) or “nitrosative stress” (NS, induced by RNS), which is directly linked with organismal fitness and cellular/organismal senescence. Since their discovery in the 1950s, these particles have been described as extremely harmful compounds which, among other things, are responsible for cellular damage, mutagenesis and ageing. The effects of abiotic factors (e.g. changing environmental conditions or the presence of pollutants) on FR formation, has been extensively studied for a wide variety of organisms. However, the effects of changes in environmental salinity have been mainly addressed in plants, while in the animal kingdom, studies concentrate on vertebrate species. Thus, there is an important gap of knowledge in how invertebrate species, and especially fascinating models such as meiofauna (eg. platyhelminths), physiologically handle such daily changes and how these affect FR production. Furthermore, the exact mechanism of induction of OS or NS by salinity remains unclear (Paital and Chainy, 2012).
But the ROS and RNS formation that accompanies stress should necessarily be viewed as s harmful event which need to be avoided or aleviated?
The answer is NO. ROS and RNS can also be viewed as a perquisite for the organism to adequately respond and induce proper acclimation mechanisms (Miller et al., 2010). Since the beginning of the 21st century, FRs have been reported by many authors to be involved in important physiological functions, such as muscle relaxation, immune reactions, signal transduction, apoptotic cell death, homeostasis and induction of adaptive mechanisms (reviewed by Turrens, 2003). One good example is the case of nitric oxide (NO): NO is a highly diffusible gas which has a great variety of physiological functions, including suppression of cellular oxygen consumption (Moncada & Erusalimsky, 2002) by changing the oxygen affinity of cytochrome c oxidase (the terminal enzyme in the mitochondrial electron transport chain). Thus, it has been suggested that free radicals such as NO can be involved in the physiological changes leading to hypoxic adaptation. NO stabilizes under hypoxic conditions and has also been suggested to mediate metabolic rate depression in some marine bivalves below the low critical pO2 (pc) and to be involved in the switch from oxy-regulation to oxy-conformity (Strahl and Abele, submitted). I have myself demonstrated that the applies for the intertidal platyhelminth M. lignano (Rivera-Ingraham et al., 2013a). Taking into account that NO can also be produced from NO2 under acidic conditions and that the half-life of NO in aqueous solutions is significantly higher at low pO2 (<10 kPa) than in normoxic conditions (21kPa), NO can be also considered as a promising candidate involved in hypoxia signaling in marine invertebrates.Further, on the hyperoxic side of the spectrum of environmental oxygenation, it has been suggested that changes in the environmental H2O2 levels may play a major role as an external signal of too high oxygen levels in marine animal ectotherms. Environmental H2O2 levels were shown to reduce respiration rates in the polychate Nereis diversicolor (Abele-Oeschger et al., 1994), the shrimp Crangon crangon (Abele-Oeschger et al., 1997) and the limpet Nacella concinna (Abele et al 1998), presumably by disturbing energy consuming processes such as active (ATP consuming) membrane transporters.But compared to the effect and signaling role of ROS/RNS in hypoxia adaptations, the knowledge applied to the effect of salinity has been greatly unattended in marine organisms. Most of the knowledge regarding the signaling role of certain ROS/RNS in the adaptive response to salinity changes, comes from plant biology (reviewed by Miller et al., 2010), and very little information is available for animal cells. NO has been recognized as an important endogenous signaling molecule that orchestrates multiple defense responses to both abiotic and biotic stress in plants (Neill et al., 2003). Several other experiments evidence that NO serves as a signal in inducing salt tolerance by regulating Na+ homeostasis and K+ acquisition via increased expression of plasma membrane Na+/H+ antiporter and H+-ATPase-related genes (Zhang et al., 2006). H2O2 has also been proven to act as a signaling molecule in salinity tolerance (Pletjushkina et al., 2006; Slesak et al., 2007) as it may activate Ca2+-channels increasing [Ca2+cyt] in plant cells (Pei et al., 2000), and many studies have revealed that transient [Ca2+cyt] fluctuation plays an essential signaling role in many stress responses (Hung et al., 2005).
However, in order for FRs to act as intercellular signals, these must cross at least two membranes:
(i) the plasma membrane of the compound-producing cell and
(ii) the plasma membrane of the compound signal-perceiving cell. But little is known about their transport from the site or origin to the place of action.
For the specific case of H2O2, it has been recently shown that this molecule can diffuse through specific members of the aquaporin (AQP) family (Bienert et al., 2007), which are, in turn, key channels involved in osmoregulation. Therefore, through this project, we aim to determine the effect of salinity changes on the physiology of our model organisms and how these changes affect the production of free radicals. Are certain ROS or RNS involved in triggering physiological adaptations to changes in water salinity and are AQPs facilitanting their diffusion and possible signalling role? Check our research questions and hypothesis here.